The Nigerian government has proposed a legally binding net-zero greenhouse gas emissions target by 2060 after the Paris Agreement in Glasgow in 2015, hence the country's need to introduce renewable/clean energy options. After COP 21, Nigeria’s Nationally Determined Contributions (NDC) highlight various goals, among which include a 20% reduction in GHG emissions by 2030 and a conditional reduction of 47% as compared to the current emission level by 2030 and expanding and diversifying sustainable energy sources to reduce the vulnerability of fossil fuels to climate impacts. This prompted the country to create the National Renewable Energy and Efficiency Policy (2022), part of which is a “30:30:30” plan. By 2030, it aims to add 30 GW of power output, with renewables making up 30% of the country’s energy mix.
In November 2023, the COP28 agreement advised nations to phase out fossil fuels entirely by 2060. However, despite potential energy market opportunities, Nigeria needs sizeable renewable power plants besides hydropower. Key challenges include ineffective/inefficient bureaucratic structure, lack of detailed resource assessment data, knowledge gaps, and lack of expertise. To address these, a comprehensive resource campaign is needed to assess the potential of other renewable energy options. This insight aims to discuss the potential of geothermal energy as a renewable energy option in Nigeria, its market size, and recommendations on the possibility of utilising the resource.
Nigeria’s Electricity Demand and Consumption
With a rapidly increasing human population, Nigeria has a growing demand for energy, and approximately 65 million people lack access to electricity. Statista reported that gas plants generate more than 79% of Nigeria's electricity (Fig. 1), while other renewable energy sources account for just about 21%, with hydropower accounting for the highest share at 20%.
According to Fortune Business Insights, the global geothermal energy market had a value of $66.24 billion in 2023 and is expected to increase to USD 70.14 billion in 2024. They reported a compound annual growth rate (CAGR) of 6.61% over a forecast period, and the market is projected to reach USD 179 billion by 2030 (Fig. 2). In 2023, the Asia Pacific region held the largest share of the geothermal energy market, accounting for 37.14%.
As an African energy giant, Nigeria needs to up its game and explore this renewable energy option to tap into the market opportunities.
Is Geothermal Energy a Path to Energy Decarbonisation?
The Greek words "geo," meaning earth, and "therme," meaning heat, coined the term "geothermal." Therefore, it refers to the heat that originates from within the earth. It is preferred to other renewable energy sources due to its constant availability, as it is not reliant on weather conditions. The sketch in Figure 3 shows how the earth's internal heat originates from the molten magma, which is about 3000 km beneath the earth. Subsurface radioactive processes can also generate heat. This is known as radiogenic heat. The major heat-producing elements in rock minerals are potassium (K), uranium (U238), and thorium (Th). Heat within the earth can be used for electricity generation. When a well is drilled into the earth to capture the rising hot water or steam, we can use this heat from within the earth to generate electricity. The earth transfers heat through conduction, convection, and radiation. This heat, in the form of steam or hot fluid, drives the turbines to produce electricity. Most of the countries with geothermal energy facilities employ dry-steam systems for power generation.
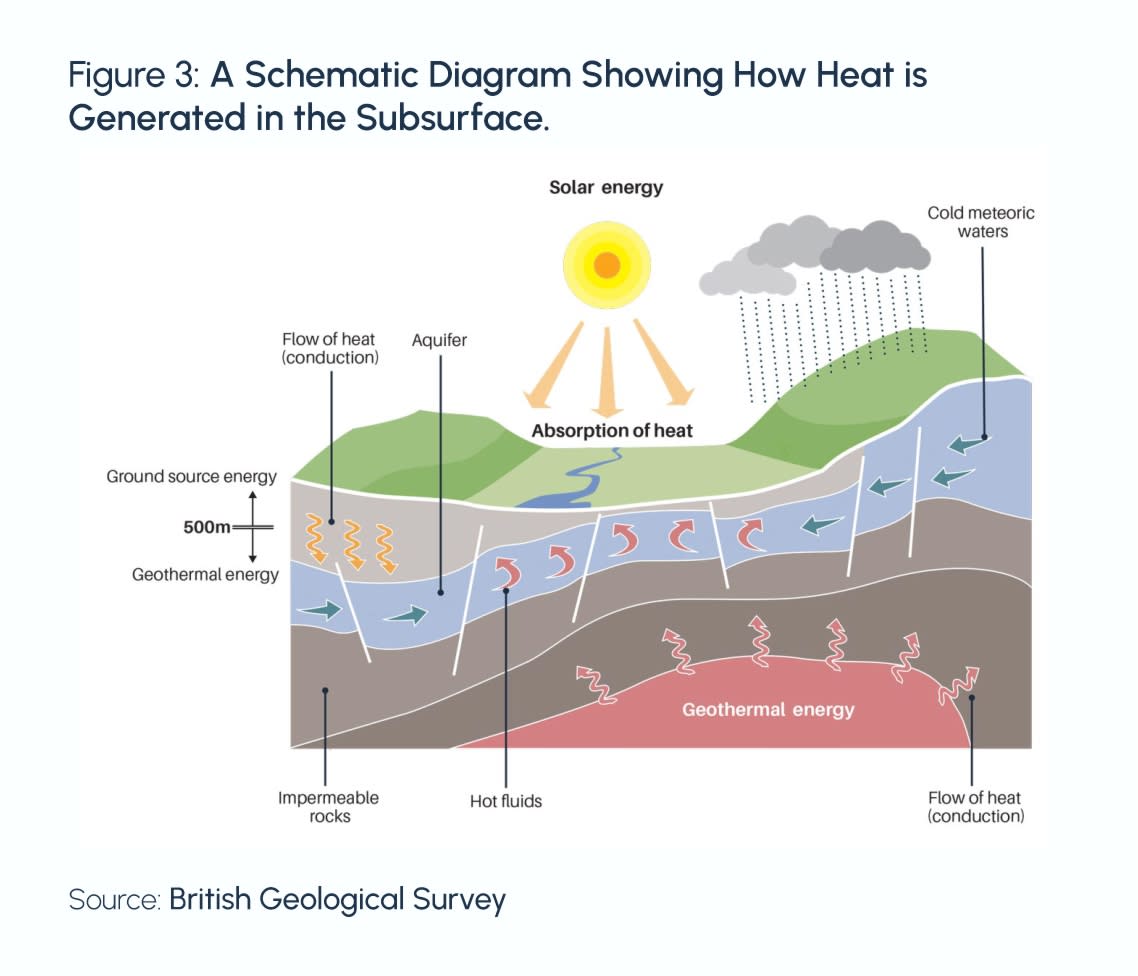
Geothermal energy is visible on the surface in the form of warm lakes and springs, volcanoes, and geysers. Hot or warm springs are the most common geothermal features in Nigeria, where there are no active plates and volcanoes like in Kenya, the USA, Italy, and so on.
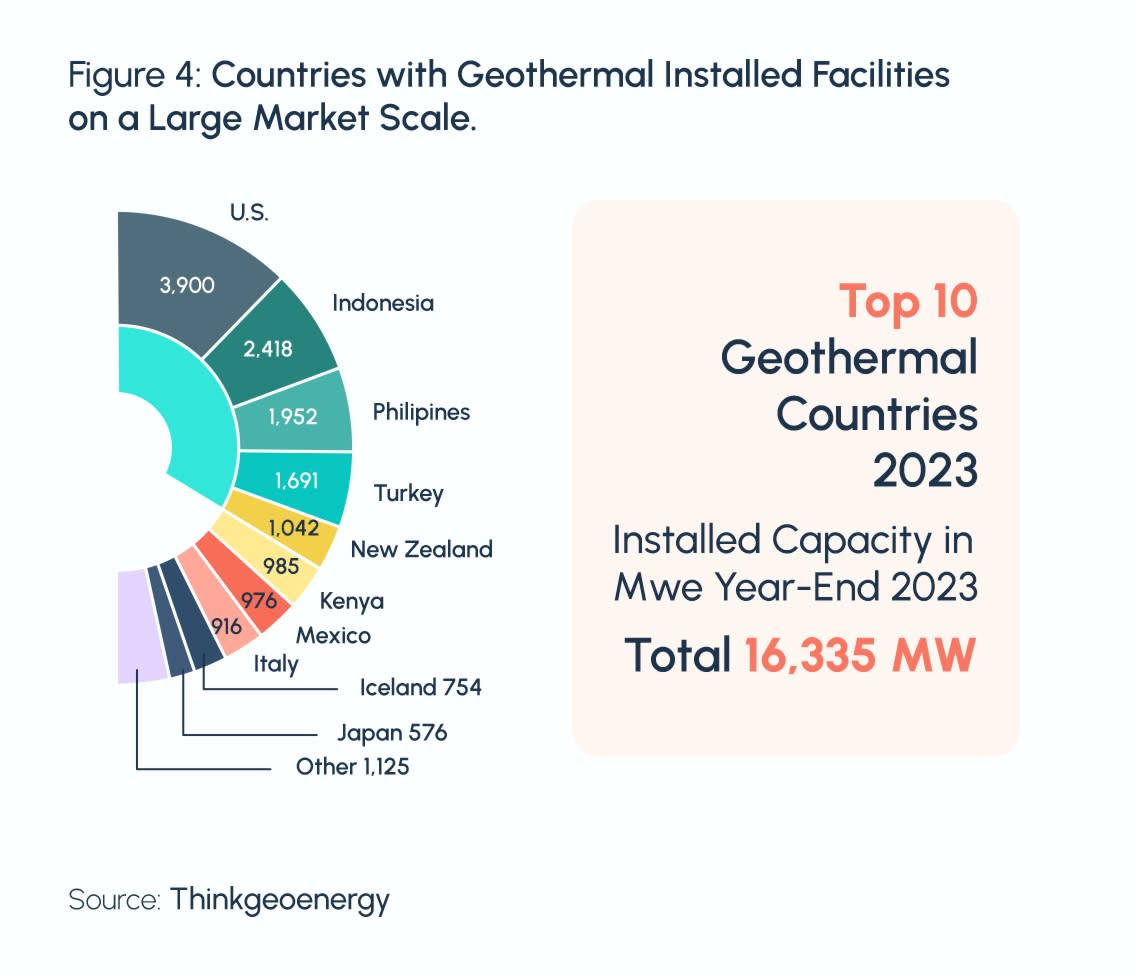
According to Thinkgeoenergy, there is a total installed capacity of 16.3 gigawatts (GW) in 24 countries as of 2023, and Figure 4 shows the top 10 geothermal countries around the world. Geothermal energy is utilised for heating purposes in 65 countries, whereas 21 countries employ it for generating both electrical and thermal power. The worldwide potential of geothermal energy for the production of electricity is currently estimated to be 4.9 gigawatts (GWe), and it is consistently increasing at an annual pace of approximately 200 megawatts (MW). One megawatt hour of geothermal power can result in an approximate offset of one ton of carbon dioxide gas emissions if replaced with one-megawatt hour of fossil fuel power plants.
Nigeria’s Potential: Geological Suitability and Resource Exploration
The electricity generation from geothermal power plants depends entirely on the area's geology. Geologists categorise Nigeria into three primary groups: the sedimentary basins, the basement complex rocks, and the younger granites. The basement complex underlies the sedimentary basins. The Basement Complex consists of rocks that have a crystalline origin and can be further categorised into three types: migmatite-gneiss, Schist Belts, and Older Granites. The majority of these rocks from the basement complex are located in the north-central region of Nigeria. The Nigerian Basement Complex covers approximately 50% of the country's total land area.

In a non-volcanic region like Nigeria, geothermal resources can only be sourced from a high geothermal gradient, primarily due to the spontaneous decay of the naturally occurring radioactive isotopes potassium-40, uranium-238, and thorium-232, which are mainly located in the mantle (Barbier, 2002).
Known geothermal exploration sites in Nigeria are the Lamurde hot spring, also known as “ruwan zafi” in Adamawa State, the Ikogosi Warm Springs of Ondo State, and the Wikki Warm Springs of Bauchi State. This is a great indicator of possible hydrothermal systems or hot sedimentary aquifers. These hot aquifers are formed as a result of the radiogenic or magmatic heating of groundwater and are often used for district heating. Only a limited amount of expert research exists regarding the assessment of geothermal energy resources. Although restricted to the sedimentary basins inside the country, a reliable source of rock subsurface temperature can be obtained from the oil and gas industry (Eyinla et al., 2016).
Kwaya et al. conducted exclusive research on geothermal gradient and heat flow in the Nigerian flank of the Chad basin. They analysed the subsurface temperature data from 19 cat wells and 24 water wells, and the results revealed a geothermal gradient range of 2.81 °C/100 m to 5.88 °C/100 m with an average of 3.71 °C/100 m.
According to Brimmo .‘s research on sustainable energy development in Nigeria, Nigeria's geothermal gradient varies from 0.82 to 7.6 °C/100 m in the Chad basin, Niger Delta, and Anambra basins, with the highest gradient being observed in the northeastern region of the Niger Delta and Sokoto basins. The average value across the country is between 2 and 3 °C/100 m. This suggests that achieving the ideal temperature of 135 degrees Celsius (according to the Handbook of Best Practices for Geothermal Drilling, Albuquerque, New Mexico, 2010) for geothermal power plants requires drilling at a significantly deeper depth of approximately 5000 m to 7000 m. These temperature values were derived from well data collected during oil and gas exploration in the sedimentary basins of Nigeria.
Nigerian basement complex terrain has not been explored much and lacks sufficient subsurface temperature data due to a lack of hydrocarbons therein. However, it consists mainly of gneisses and granites, which have very good thermal conductivity (Fig. 6) and can be efficient for heat transfer.
Figure 6: Thermal Conductivity of Different Rocks and Fluids
Source: Bucher 2021
Geothermal Restraint
Every natural resource requires exploration and development, which is always at the high end. In the initial years, the cost of drilling wells, taking landsat images, geochemical analysis, reservoir simulation, and engineering all affected the cost of production, making the cost of research and development very high. Also, geothermal well design closely resembles that of hydrocarbon production, with the exception that it typically involves a production-injection well pair, which can be quite costly. This accounts for nearly 70% of the project cost. Next is the issue of limited geothermal data, especially in the potential sites. Researchers and developers must identify the best location to make the resource have as close to zero carbon footprint as possible. A very common and readily available renewable option is the use of solar energy to turn gas turbines instead of using conventional fossil fuels. This poses a higher cost of energy production to run the power plant since solar installation is quite expensive. In addition, the energy system is still new, so the cost of expertise is another important aspect to consider. People need prior operational experience or training to optimise performance. These variables contribute to the increased expenses of installing geothermal power facilities. The excessive cost will probably hinder the growth of the market by restraining the effective utilisation of geothermal power.
Conclusions
The nation’s geothermal energy resources are currently not developed due to the unquantified potential and resource data for geothermal energy. It is advised that the federal government invest in geothermal exploration to compile a comprehensive database on Nigeria's potential for geothermal energy. This will reveal potential sweet spots for the deployment of geothermal power plants.
References
Barbier, E. (2002). Geothermal energy technology and current status: an overview. Renewable & Sustainable Energy Reviews, 6(1–2), 3–65. https://doi.org/10.1016/s1364-0321(02)00002-3
Brimmo, A. T., Sodiq, A., Sofela, S., & Kolo, I. (2017). Sustainable energy development in Nigeria: Wind, hydropower, geothermal and nuclear (Vol. 1). Renewable & Sustainable Energy Reviews, 74, 474–490. https://doi.org/10.1016/j.rser.2016.11.162
Eyinla, Dorcas & Oladunjoye, M. & Ogunribido, Tolulope & Odundun, Olumuyiwa. (2016). An Overview of Geothermal Energy Resources in Nigeria. Environtropica. 12. 61-71.
Kwaya, M. Y., Kurowska, E., & Arabi, A. S. (2016). Geothermal Gradient and Heat Flow in the Nigeria Sector of the Chad Basin, Nigeria. Computational Water Energy and Environmental Engineering, 05(02), 70–78. https://doi.org/10.4236/cweee.2016.52007
Kurowska E. and Schoeneich K. (2010). Proceedings World Geothermal Congress 2010 Bali, Indonesia, 25-29 April 2010.
O.O. Babalola High-potential geothermal energy resource areas of Nigeria and their geologic and geophysical assessment. AAPG Bull, 68 (6) (1984), p. 450.
Rahaman, M.A. (1988). Recent Advances in the Study of the Basement Complex of Nigeria. In Precambrian Geology of Nigeria, Geological Survey of Nigeria, Kaduna South, 11-43.
Stober, I., & Bucher, K. (2021). Geothermal Energy. In Springer eBooks. https://doi.org/10.1007/978-3-030-71685-1
Abbreviations
GW- gigawatts
TWh- terawatt hour
MW- megawatt